4.2 How Glaciers Work
There are two main types of glaciers. Continental glaciers (a.k.a. ice sheets) cover vast areas of land and only exist now in extreme polar regions, including Antarctica and Greenland (Figure 4.2.1). Alpine glaciers (a.k.a. valley glaciers) originate on mountains, mostly in temperate and polar regions, but even in tropical regions if the mountains are high enough. They are typically confined to valleys.
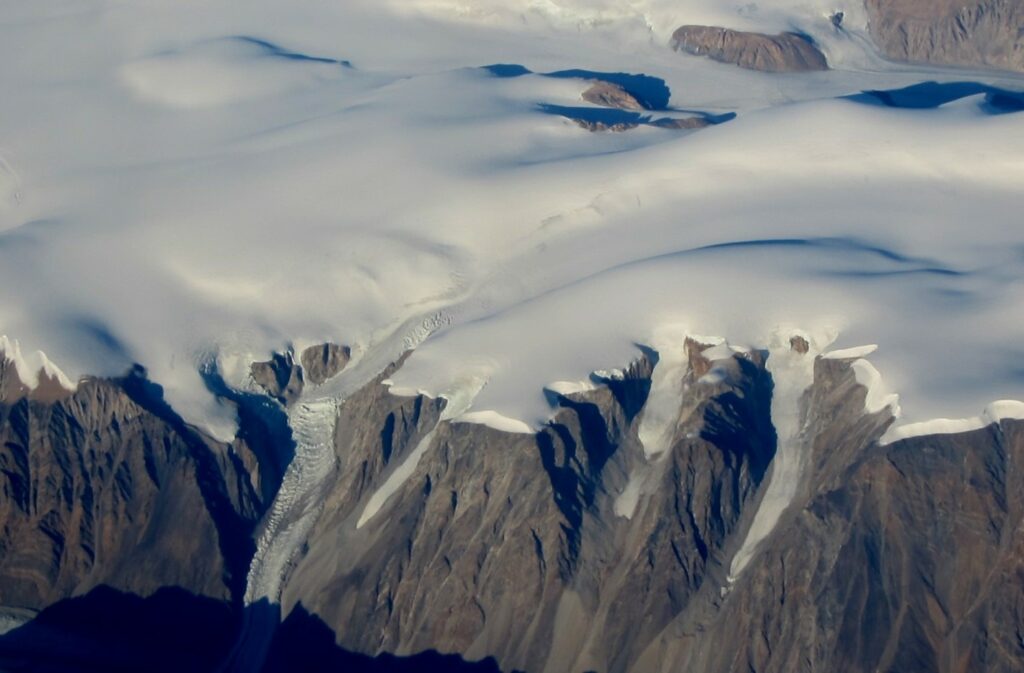
The Earth’s two great continental glaciers, on Antarctica and Greenland, comprise about 99% of all of the world’s glacial ice, and approximately 68% of all of the Earth’s fresh water. As is evident from Figure 4.2.2, the Antarctic ice sheet is vastly bigger than the Greenland ice sheet; it contains about 17 times as much ice. If the entire Antarctic ice sheet was to melt, sea level would rise by about 80 m and almost all of the Earth’s major cities would be submerged.
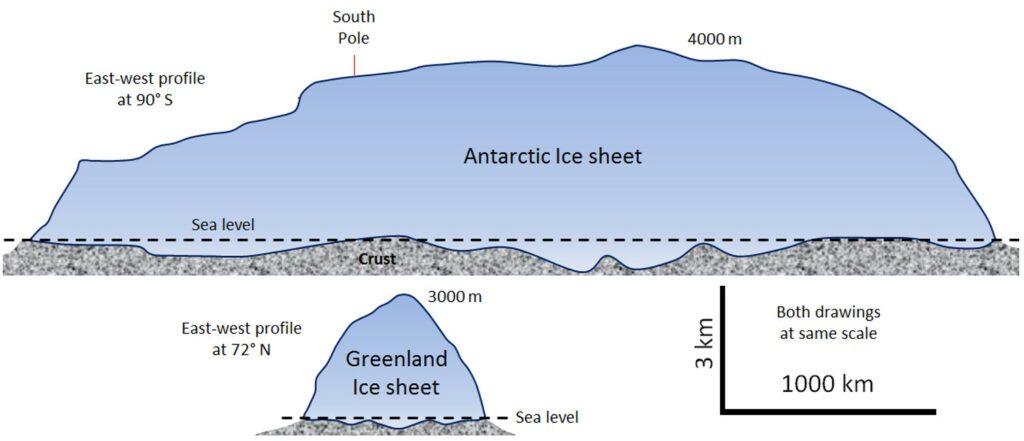
Continental glaciers do not flow “downhill” because the large areas that they cover are generally flat. Instead, ice flows from the region where it is thickest towards the edges where it is thinner. This is shown schematically on Figure 4.2.3. It means that in the central thickest parts the ice flows almost vertically down towards the base, while in the peripheral parts it flows outwards towards the margins. In continental glaciers like Antarctica and Greenland, the thickest parts (4000 and 3000 m respectively) are the areas where the rate of snowfall (and therefore of ice accumulation) are highest.
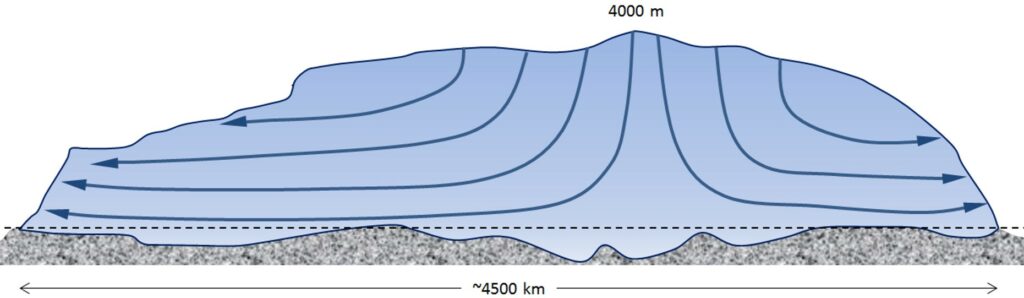
The flow of alpine glaciers is primarily controlled by the slope of the land beneath (Figure 4.2.4). In the zone of accumulation the rate of snowfall is greater than the rate of melting. In other words, not all of the snow that falls each winter melts during the following summer, and the ice surface is always covered with snow. In the zone of ablation more ice melts than accumulates as snow. The equilibrium line marks the boundary between the zones of accumulation and ablation.
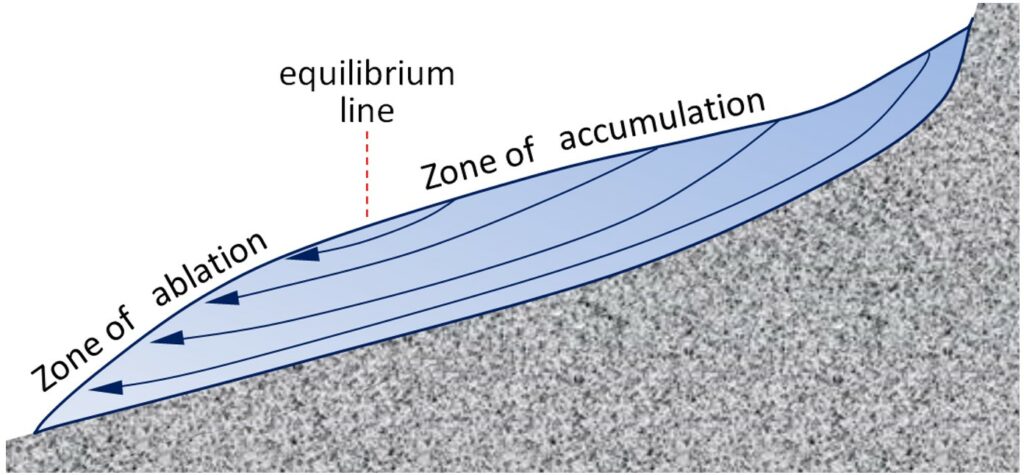
The equilibrium line of the Overlord Glacier, near to Whistler BC, is shown in the photo on Figure 4.2.5. Below that line, in the zone of ablation, bare ice is exposed because last winter’s snow has all melted; above that line the ice is still mostly covered with snow from the last winter. The position of the equilibrium line changes from year to year as a function of the balance between snow accumulation in the winter and snow-melt during the summer. More winter snow and less summer melting obviously favours the advance of the equilibrium line (and of the glacier’s leading edge), but of these two variables it is the summer melt that matters most to a glacier’s budget. Cool summers promote glacial advance and warm summers promote glacial retreat.
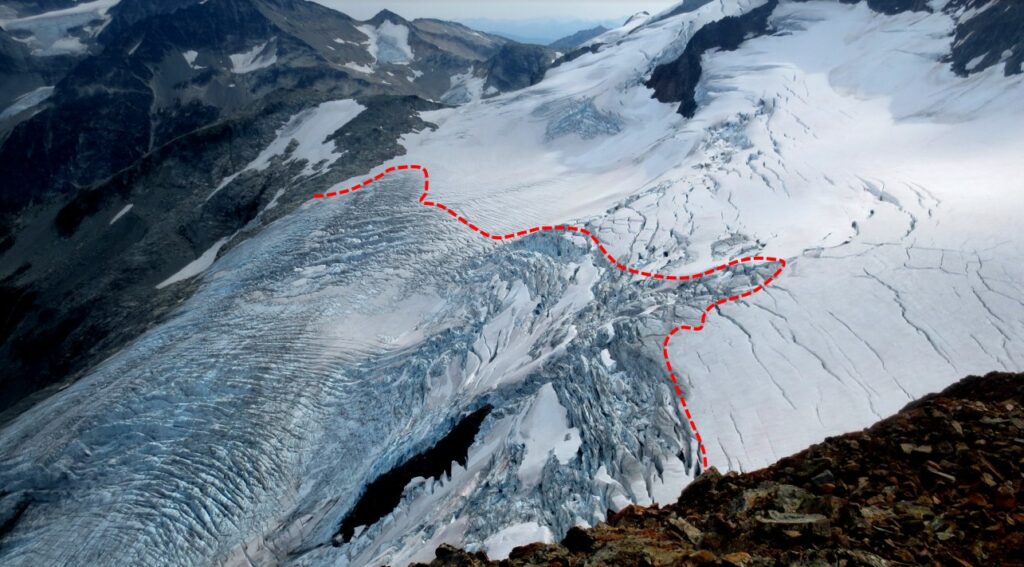
Above the equilibrium line of a glacier not all of the winter snow melts in the following summer, and thus snow gradually accumulates. The snow layer from each year is covered and compacted by subsequent snow and it gradually gets compressed and turned into firn within which the snowflakes lose their delicate shapes and become granules. With more compression the granules are pushed together and air is squeezed out. Eventually the granules are “welded” together to create glacial ice. Downward percolation of water, from melting taking place at surface, contributes to the process of ice formation (Figure 4.2.6).
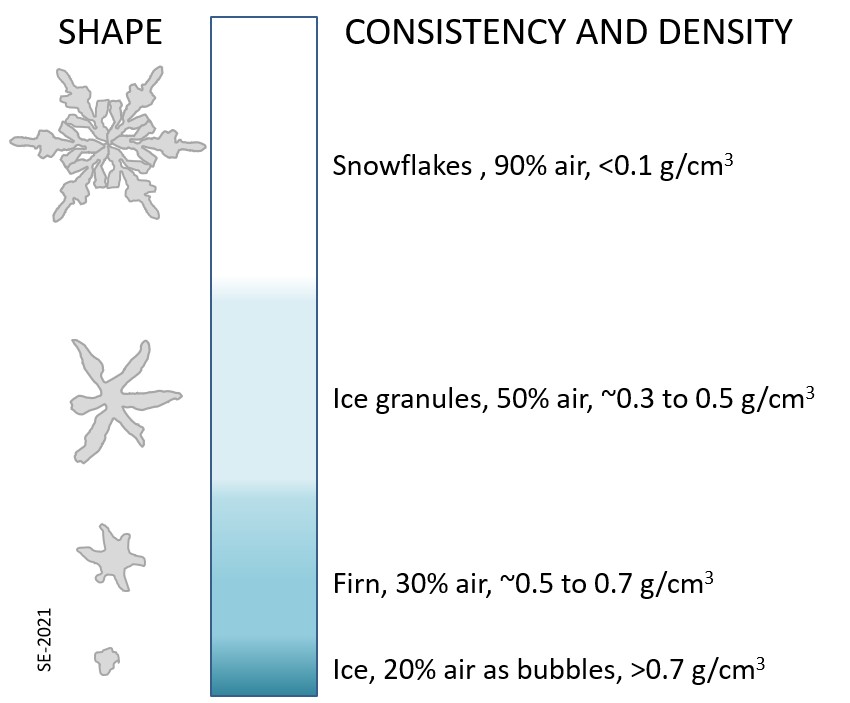
Glaciers move because the surface of the ice is sloped. This generates a stress on the ice, which is proportional to the slope and the depth below the surface. As shown on Figure 4.2.7, the stresses are quite small near to the ice surface but much larger at depth, and also greater in areas where the ice surface is relatively steep. Ice will deform, meaning that it will behave in a plastic manner, at stress levels of around 100 kilopascals, and so it’s evident that, in this case, the upper 50 to 100 m of the ice (above the dashed red line) is not plastic (it is rigid) while the lower ice is plastic and will flow. The rigid layer will be thinner where the ice surface is steeper and thicker where it is flatter.
When the lower ice of a glacier flows it moves the upper ice along with it, so although it might seem from the stress patterns (red numbers and red arrows) shown on Figure 4.2.7 that the lower part should move the most, in fact while the lower part deforms (and flows) and the upper part doesn’t deform at all, the upper part moves the fastest because it is pushed along by the lower ice.
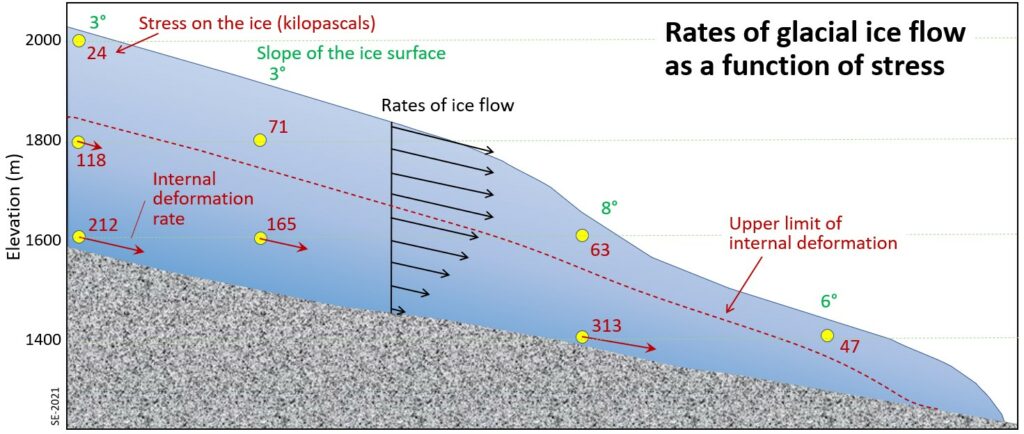
The plastic lower ice of a glacier can flow like a very viscous fluid, and it can therefore flow over irregularities in the base of the ice, and also around corners. But the upper rigid ice cannot flow in this way, and because it is being carried along by the lower ice it tends to crack where the lower ice has to flex. This leads to the development of crevasses in areas where the rate of flow of the plastic ice is changing. In the area shown on Figure 4.2.8, for example, the glacier is speeding up over the steep terrain, and the rigid surface ice has to crack to account for the change in velocity.
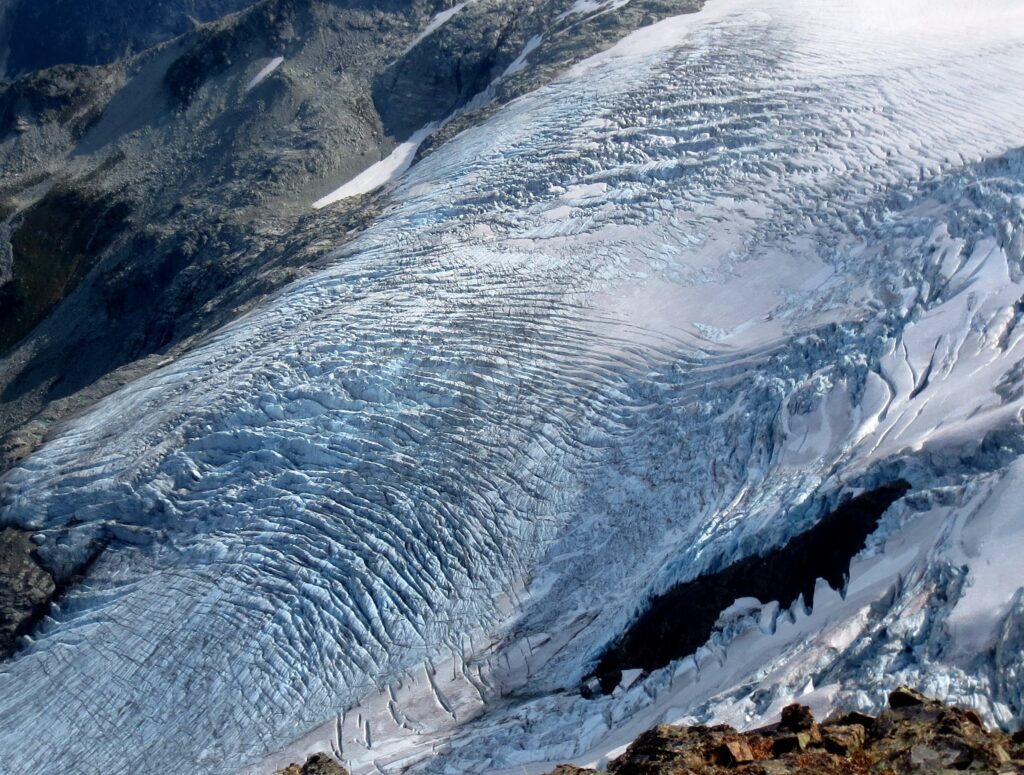
The base of a glacier can be cold (below the freezing point of water) or warm (above the freezing point). If it is warm there will likely be a film of water between the ice and the material underneath, and the ice will be able to slide over that surface. This is known as basal sliding (Figure 4.2.9, left). If the base is cold the ice will be frozen to the material underneath and it will be stuck—unable to slide along its base. In this case all of the movement of the ice will be by internal flow (Figure 4.2.9, right).
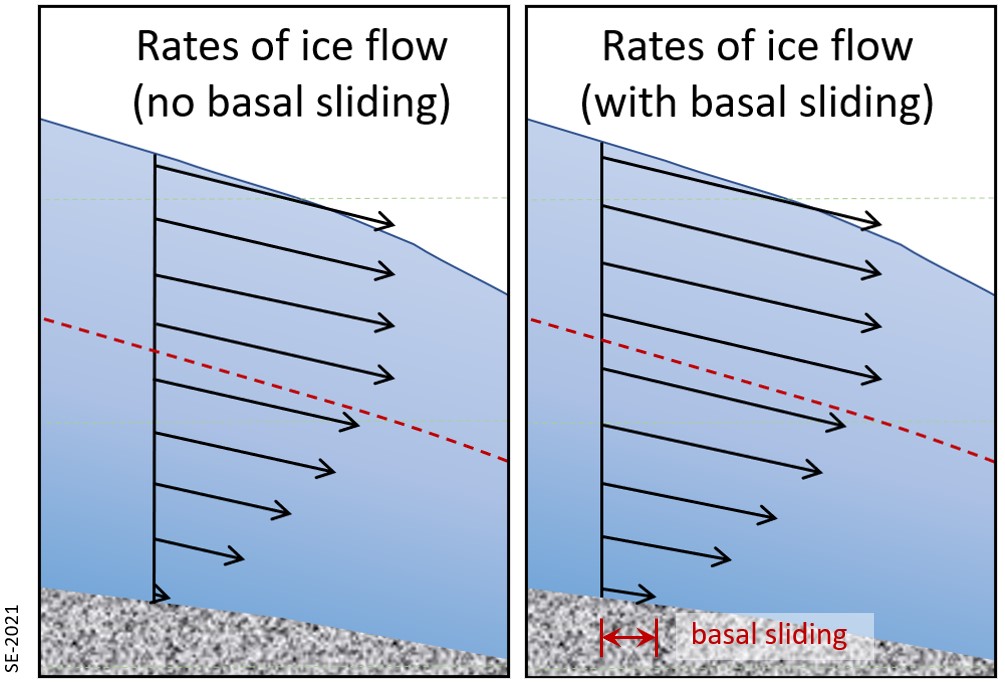
One of the factors that affects the temperature of the base of a glacier is the thickness of the ice. Ice is a good insulator. The slow transfer of heat from the Earth’s interior will provide enough heat to warm up the base if the ice is thick, but not enough if it is thin and that heat can escape. It is typical for the leading edge of an alpine glacier to be relatively thin (see Figure 4.2.7), and so it is common for that part to be frozen to its base while the rest of the glacier is still sliding. This is illustrated on Figure 4.2.10 for the Athabasca Glacier. Because the leading edge of the glacier is stuck to its frozen base, while the rest continues to slide, the ice coming from behind has pushed (or thrust) itself over top of the part that is stuck fast.
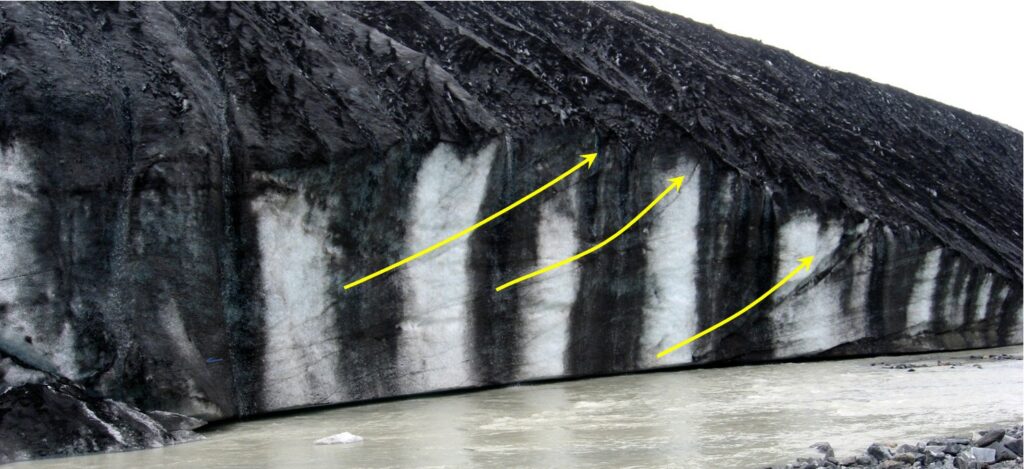
Just as the base of a glacier moves slower than the surface, the edges, which are more affected by friction along the sides, move slower than the middle. If we were to place a series of markers across an alpine glacier and come back a year later we would see that the ones in the middle have moved further forward than the ones near to the edges (Figure 4.2.11).
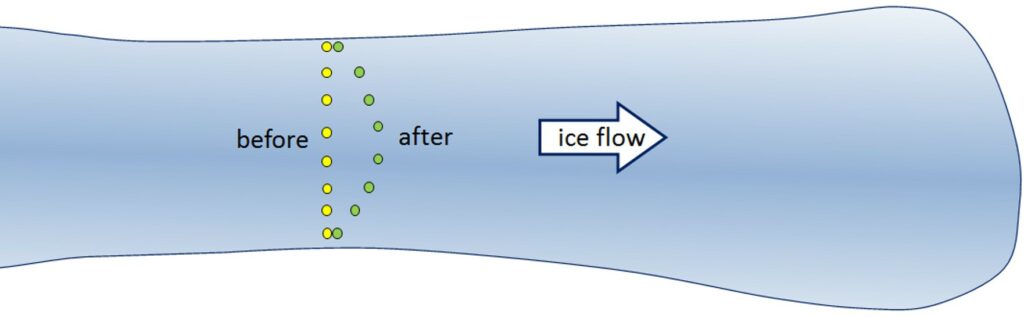
Glacial ice always moves downhill (or down from an area of thicker ice in the case of continental glaciers), in response to gravity, but the front edge of a glacier is almost always melting or else calving into water (shedding icebergs). Alpine glaciers can flow up over bumps in the terrain if the ice is thick enough. If the rate of forward motion of the glacier is faster than the rate of ablation (melting) the leading edge of the glacier will advance (move forward). If the rate of forward motion is about the same as the rate of ablation, the leading edge will remain stationary, and if the rate of forward motion is slower than the rate of ablation, the leading edge will retreat (move backward). Even if a glacier is retreating, the ice of the glacier will be moving forward.
Calving of icebergs is an important process for glaciers that terminate in lakes or the ocean. An example is shown on Figure 4.2.12.
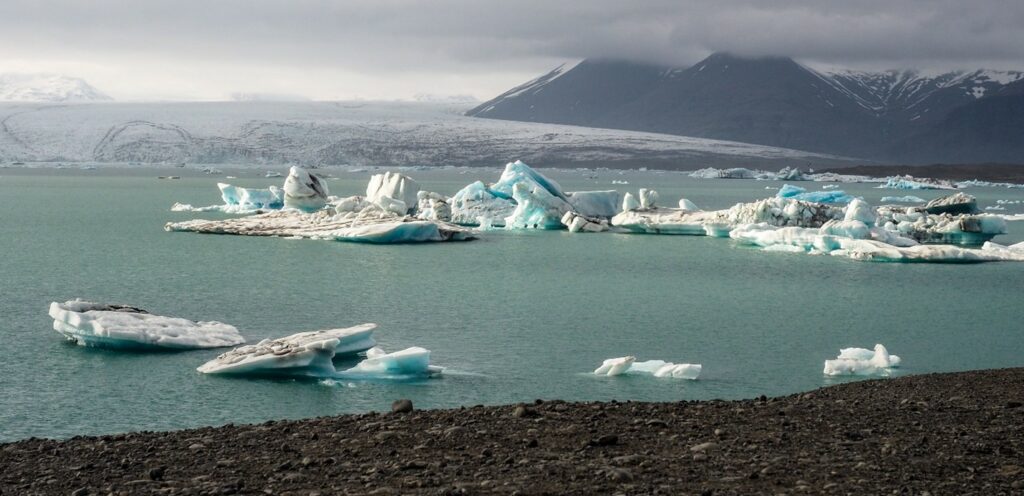
Exercise 4.2 Moving Ice
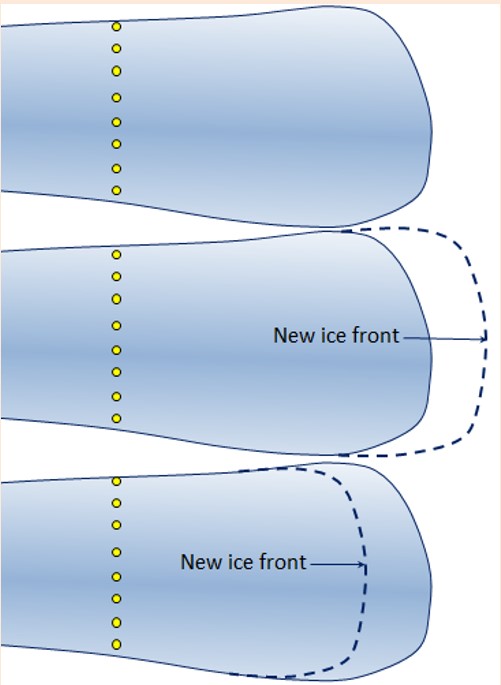
Figure 4.2.13 represents a glacier onto the surface of which some markers (yellow dots) have been placed to determine the rate of ice motion over a one-year period. The ice is flowing from left to right.
In the middle diagram the leading edge of the glacier has advanced. Draw in where the markers might have moved to.
In the lower diagram the leading edge of the glacier has retreated. Draw in where the markers might have moved to.
Exercise answers are provided Appendix 2
Media Attributions
- Figure 4.2.1 Photo by Ginny McLean, used with permission, CC BY 4.0
- Figure 4.2.2 Steven Earle, CC BY 4.0
- Figure 4.2.3 Steven Earle, CC BY 4.0
- Figure 4.2.4 Steven Earle, CC BY 4.0
- Figure 4.2.5 Modified by Steven Earle, CC BY 4.0, from a photo by Isaac Earle, 2013. Used with permission.
- Figure 4.2.6 Steven Earle, CC BY 4.0
- Figure 4.2.7 Steven Earle, CC BY 4.0
- Figure 4.2.8 Photo by Isaac Earle, used with permission, CC BY 4.0
- Figure 4.2.9 Steven Earle, CC BY 4.0
- Figure 4.2.10 Steven Earle, CC BY 4.0
- Figure 4.2.11 Steven Earle, CC BY 4.0
- Figure 4.2.12 Photo by Isaac Earle, 2015, used with permission, CC BY 4.0
- Figure 4.2.13 Steven Earle, CC BY 4.0