10.2 Chemical Weathering
Chemical weathering results from the chemical changes to some minerals that become unstable when they are exposed to surface conditions. The kinds of changes that take place are highly specific to the mineral and to the environmental conditions. Some minerals, like quartz, are virtually unaffected by chemical weathering, while others, like feldspar, are quite easily altered. In general, the degree of chemical weathering is greatest in warm and wet climates, and least in cold and dry climates. The important characteristics of surface conditions that lead to chemical weathering are: the presence of water (in the air and on the ground surface), the abundance of oxygen, and the presence of carbon dioxide, which, when combined with water, produces weak carbonic acid. That process, which is fundamental to most chemical weathering, can be shown as follows:
H2O + CO2 -> H2CO3
Here we have water (e.g., as rain) plus carbon dioxide in the atmosphere, combining to create carbonic acid. The amount of CO2 in the air is enough to make only very weak carbonic acid, but there is typically much more CO2 in the soil, so water that percolates through the soil can become significantly more acidic.
There are two main types of chemical weathering. On the one hand, some minerals become altered to other minerals. For example, feldspar is altered—by hydrolysis—to clay minerals. On the other hand, some minerals dissolve completely, and their components go into solution. An example is calcite (CaCO3) which is soluble in acidic solutions.
The hydrolysis of feldspar can be written like this:
CaAl2Si2O8 + H2CO3 + ½O2 -> Al2Si2O5(OH)4 + Ca2+ + CO32-
(plagioclase + carbonic acid + oxygen -> kaolin + calcium & carbonate ions in solution)
This reaction shows calcium plagioclase feldspar, but similar reactions could also be written for sodium or potassium feldspars. In this case we end up with the mineral kaolinite along with calcium and carbonate ions in solution. Those ions can eventually combine to form the mineral calcite, and that will probably happen in the ocean. The hydrolysis of feldspar to clay is illustrated on Figure 10.2.1, which shows two images of the same granitic rock, a recently broken fresh surface on the left, and a clay-altered weathered surface on the right. Other silicate minerals can also go through hydrolysis, although the end results will be a little different. For example, pyroxene can be converted to the clay minerals chlorite or smectite, or olivine can be converted to the clay mineral serpentine.
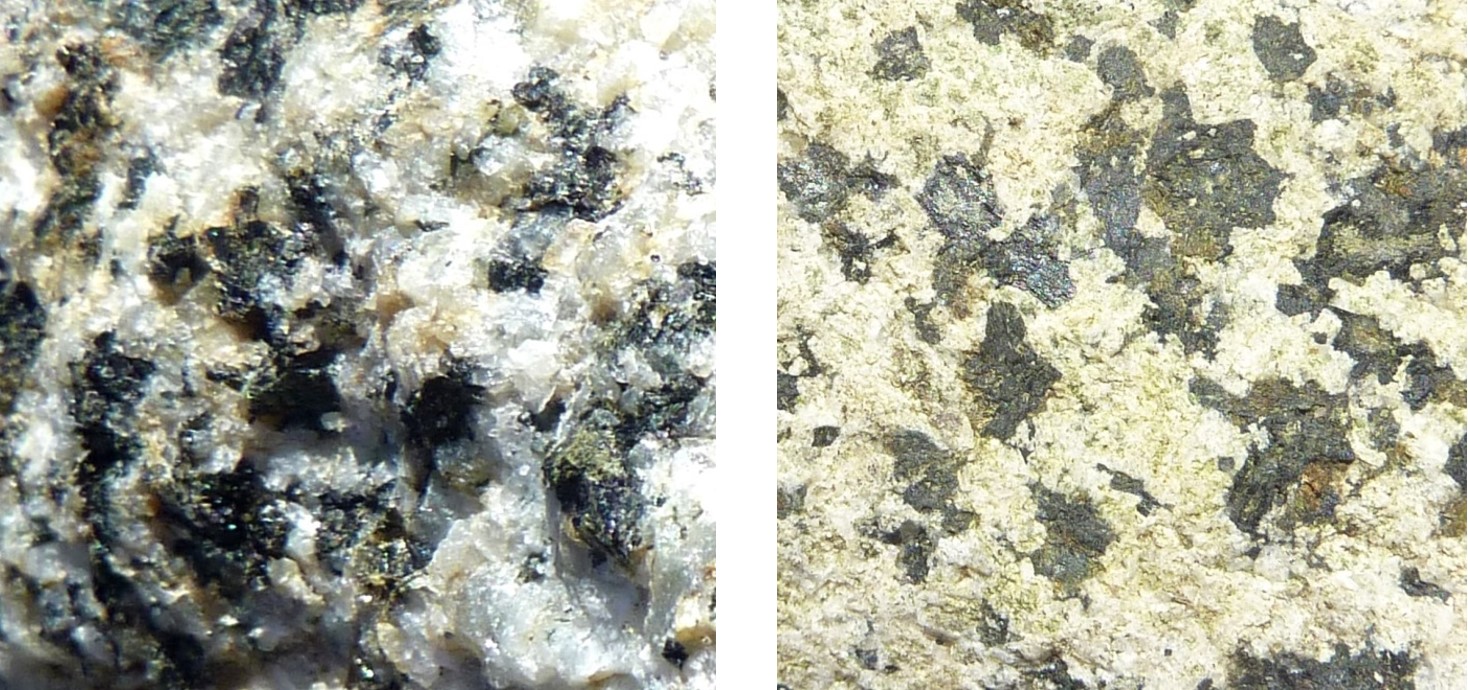
Oxidation is another very important chemical weathering process. The oxidation of the iron in a ferromagnesian silicate starts with the dissolution of the iron. For olivine the process looks like this, where olivine in the presence of carbonic acid is converted to dissolved iron, carbonate and silicic acid:
Fe2SiO4 + 4H2CO3 -> 2Fe2+ + 4HCO3– + H4SiO4
(olivine + carbonic acid -> iron, carbonate & silica ions in solution)
The equation is shown here for olivine, but could apply to almost any other ferromagnesian silicate, including pyroxene, amphibole and biotite. Iron in sulphide minerals (e.g., pyrite) can also be oxidized in this way. And the mineral hematite is not the only possible end result either, as there is a wide range of iron-oxide minerals that can form in this way. The results of this process are illustrated on Figure 10.2.2, which shows a granitic rock in which some of the biotite and amphibole have been altered to form the iron oxide mineral limonite.
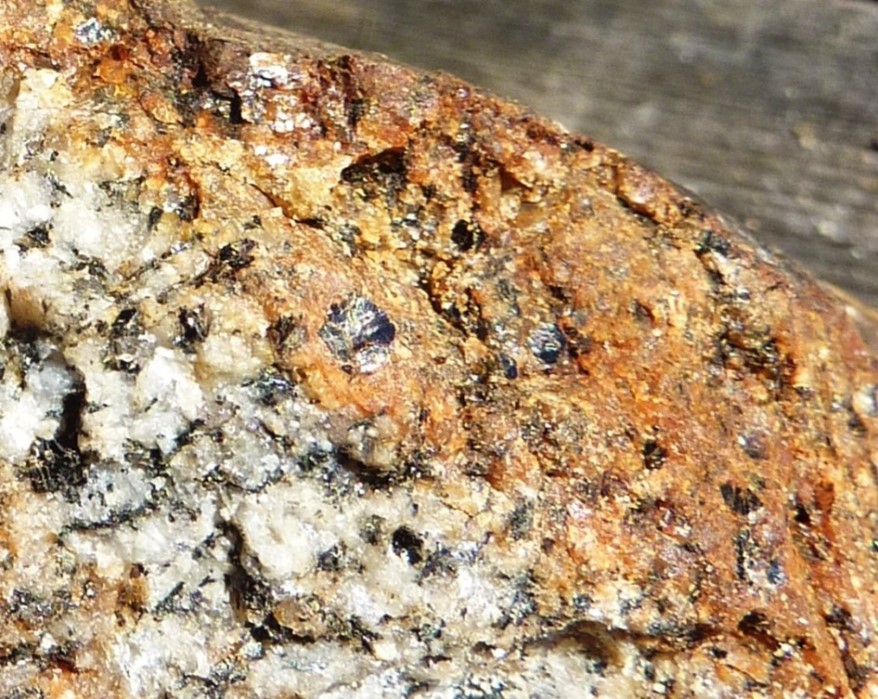
As we’ve seen above in Chapter 8, a special type of oxidation takes place in areas where the rocks have elevated levels of sulphide minerals, especially pyrite (FeS2). Pyrite will react with water and oxygen to form sulphuric acid, as follows:
2FeS2 + 7O2 + 2H2O -> Fe2+ + 2H+ + H2SO4
(pyrite +m oxygen -> iron and hydrogen ions in solution + sulphuric acid)
The runoff from areas where this process is taking place is known as acid rock drainage (ARD), and even a rock with 1 or 2% pyrite can produce significant ARD. Some of the worst examples of ARD are at metal mine sites, especially where pyrite-bearing rock and waste material has been mined from deep underground and then piled up and left exposed to water and oxygen.
The hydrolysis of feldspar, and of other silicate minerals as well, and the oxidation of iron in ferromagnesian silicates all serve to create rocks that are softer and weaker than they were to begin with, and thus more susceptible to mechanical weathering.
The weathering reactions that we’ve discussed so far involved the transformation of one mineral to another mineral (e.g., feldspar to clay), and the release of some ions in solution (e.g., Ca2+). Some weathering processes involve the complete dissolution of a mineral. Calcite, for example, will dissolve in weak acid, to produce calcium and bicarbonate ions. The equation is as follows:
CaCO3 + H2CO3 -> Ca2+ + 2HCO3–
(calcite + carbonic acid -> calcium + bicarbonate ions in solution)
Calcite is the major component of limestone (typically more than 95%), and under surface conditions limestone will dissolve to varying degrees (depending on which minerals it has other than calcite), as shown on Figure 10.2.3. Limestone also dissolves at relatively shallow depths underground, forming limestone caves. This is discussed in more detail in Chapter 14, where we look at groundwater.
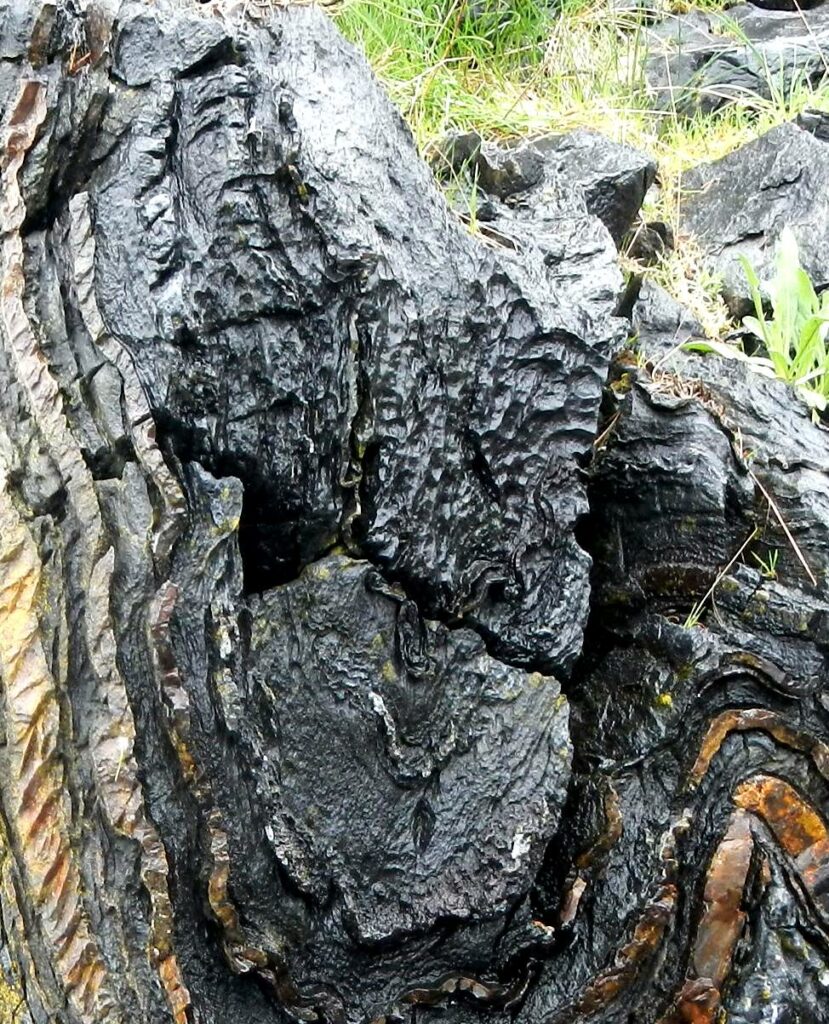
Exercise 10.2 Chemical Weathering
The main processes of chemical weathering are hydrolysis, oxidation, and dissolution. Complete the following table by indicating which process is primarily responsible for each of the described chemical weathering changes:
Exercise answers are provided Appendix 2
Products of Weathering and Erosion
The products of weathering and erosion are the unconsolidated materials that we find around us on slopes, beneath, beside and on top of glaciers, in stream valleys, on beaches, and in deserts. The nature of these materials—their composition, size, degree of sorting, and degree of rounding—is determined by the type of rock that is being weathered, the nature of the weathering, the erosion and transportation processes, and the climate.
In addition to these solid sediments, the other important products of weathering are many different types of ions in solution.
A summary of the weathering products of some of the common minerals present in rocks is provided in Table 10.1.1. In addition to the weathering products listed in the table, most of the larger fragments—larger than sand grains—that make up sediments will be pieces of rock as opposed to individual minerals.
Common Mineral | Typical Weathering Products |
Quartz | Quartz as sand grains |
Feldspar | Clay minerals plus potassium, sodium, and calcium in solution |
Biotite & amphibole | Chlorite plus iron and magnesium in solution |
Pyroxene & olivine | Serpentine plus iron and magnesium in solution |
Calcite | Calcium and carbonate in solution |
Pyrite | Iron oxide minerals plus iron in solution and sulphuric acid |
Some examples of the products of weathering are shown in Figure 10.2.4. They range widely in size and shape depending on the processes involved in their transportation. If and when deposits like these are turned into sedimentary rocks, the textures of those rocks will vary significantly. Importantly, when we describe sedimentary rocks that formed millions of years in the past, we can use those properties to make inferences about the conditions that existed during their formation.
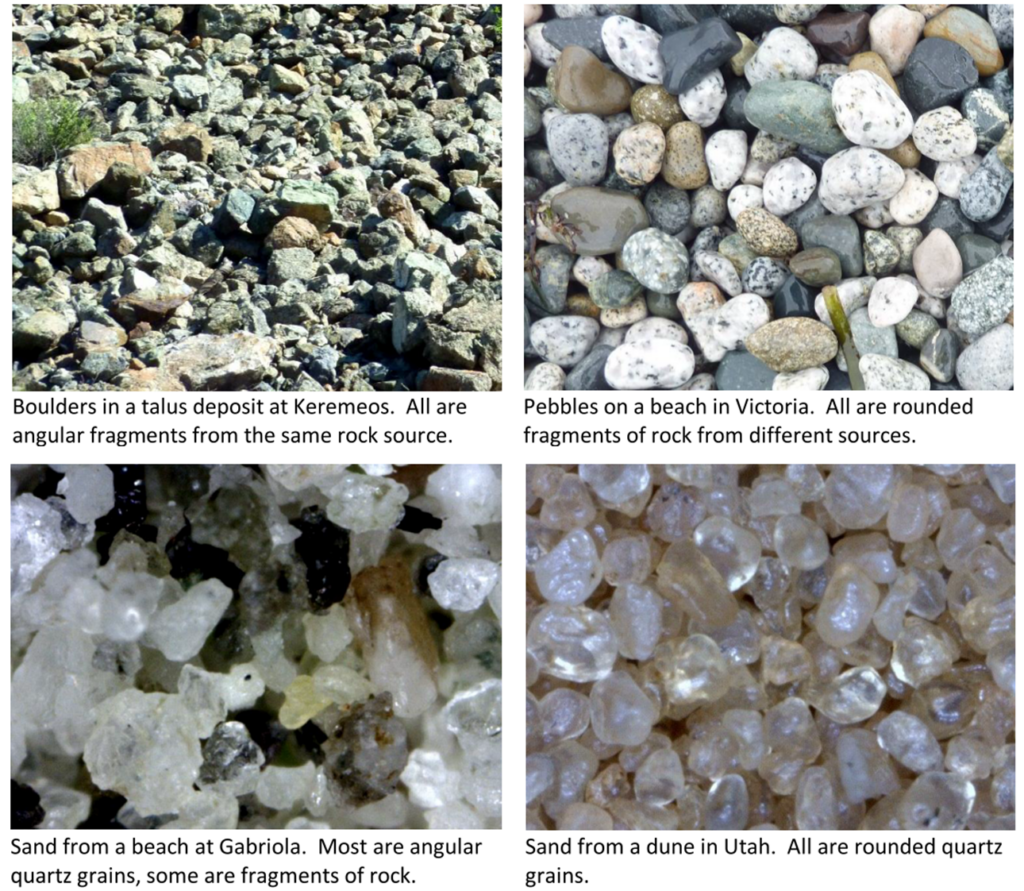
It’s worth considering here why the sand-sized sediments shown in Figure 10.2.4 are so strongly dominated by the mineral quartz, even though quartz makes up less than 20% of Earth’s crust. The explanation is that quartz is highly resistant to the weathering that occur at Earth’s surface. It is not affected by weak acids, or water or the presence of oxygen. This makes it unique among the minerals that are common in igneous rocks. Quartz is also very hard, and doesn’t have cleavage, so it is resistant to mechanical erosion.
When a rock like granite is subject to chemical weathering the feldspar and the ferromagnesian silicates get converted to clays plus dissolved ions such as: Ca2+, Na+, K+, Fe2+, Mg2+, and H4SiO4, but the quartz is resistant to those processes and remains intact. The clay gradually gets eroded away, then the rock breaks apart leaving lots of grains of quartz. In other words, quartz, clay minerals, and dissolved ions are the most common products of weathering. Quartz and some of the clay minerals tend to form sedimentary deposits on and at the edges of continents, while the rest of the clay minerals and the dissolved ions tend to be washed out into the oceans to form sediments on the sea floor.
Exercise 10.3 The Weathering Origins of Sand
A number of different sands are pictured and described in the following table. Describe some of the important weathering processes that might have led to the development of these sands.
(Photos by Steven Earle, CC BY 4.0)
Media Attributions