3.1 Changes in Solar Output and in the Earth’s Atmosphere
The solar system, which has been around just a little longer than the Earth (4.57 billion years), formed out of the gaseous and particulate remnants of one (or more) stars that had existed in this part of the galaxy and then exploded. The sun has changed significantly over that time and will continue to change in the future. The evolution of a typical star like ours is depicted on Figure 3.1.1.
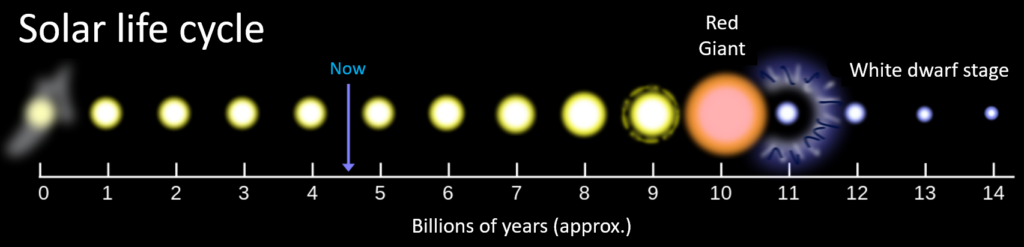
Although it’s not obvious from Figure 3.1.1, the sun’s luminosity has increased substantially over 4.57 billion years, and it is now about one-third brighter that it was originally (Figure 3.1.2). It is now producing about 33% more heat than it did at the start. This increase in intensity is a result of the ongoing conversion (by nuclear fusion) of hydrogen to helium in the sun’s core. The growing proportion of helium results in an increase in the density of the solar core region, which causes the core to contract. The increased gravitational pressure forces the hydrogen atoms closer together, and that accelerates the rate of fusion and makes the sun hotter and brighter.[1]
The sun will continue to get hotter in this way for another four billion years or so, until its core is made up entirely of helium, at which point it will evolve into a Red Giant (Figure 3.1.1) and will start to expand, first consuming Mercury, and then Venus and quite likely even the Earth. At that time the helium will start to fuse into carbon, almost half of the sun’s mass will be lost into space via massive explosions, and what remains will eventually collapse into a White Dwarf.
As noted, the solar luminosity[2] has increased by about 33% over its entire 4.6 billion year history—so far (Figure 3.1.2). That’s a huge amount from the perspective of Earth’s climate, but it’s over a very long time. The sun is going to get hotter still. Within another 4.4 billion years it will be roughly twice as hot as it was originally.
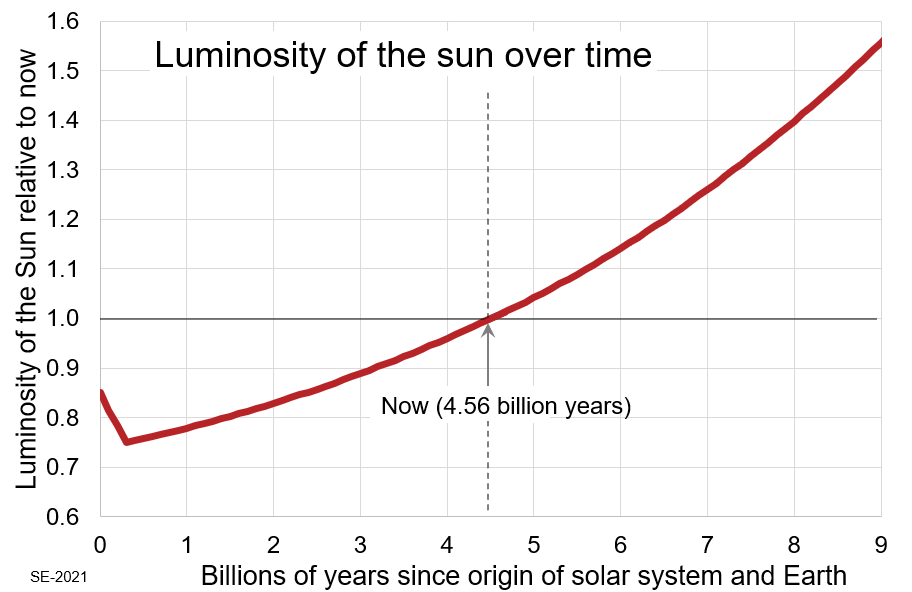
Please don’t be fooled into thinking that a warming sun is behind the climate change we see happening right now, or a reason for us to be concerned for our distant future. The present rate of solar warming is about 8% every billion years. That’s 0.008% every million years or 0.0000008% every century. That is a very, very small amount of warming on a human time scale. Over the past century it has not been enough to noticeably warm the climate—not even close. For example, the increase in luminosity due to long-term solar evolution from 1920 to 2020 was only enough to increase the Earth’s surface temperature by about 0.0000016⁰ C. During that time the surface temperature[3] has actually increased by about 1⁰ C, which is about 625,000 faster than the temperature increase that could be attributed to the change in solar luminosity over the same time period, so it’s clear that solar evolution is not the cause.
The earliest evidence of life on Earth is found in rocks about four billion years old[4]. At that time the sun was about 80% as bright as it is today. An Earth with today’s atmosphere, and with an 80% sun, would have been completely frozen (no liquid water anywhere on the surface!). That might lead us to wonder how it could have been possible for any type of life to evolve, and this is known as ‘the faint young sun paradox’. The Earth wasn’t encased in ice four billion years ago because the Archean atmosphere was very different from today’s atmosphere.[5] It was considerably thicker—approaching the density of the atmosphere of Venus—and, as shown on Figure 3.1.3, it was rich in carbon dioxide. The carbon dioxide proportion might have been in the order of 10%, as compared with today’s level of 0.04% (415 parts per million), and that provided a sufficiently strong greenhouse effect to keep the Earth’s surface temperature warm even with a relatively faint sun.
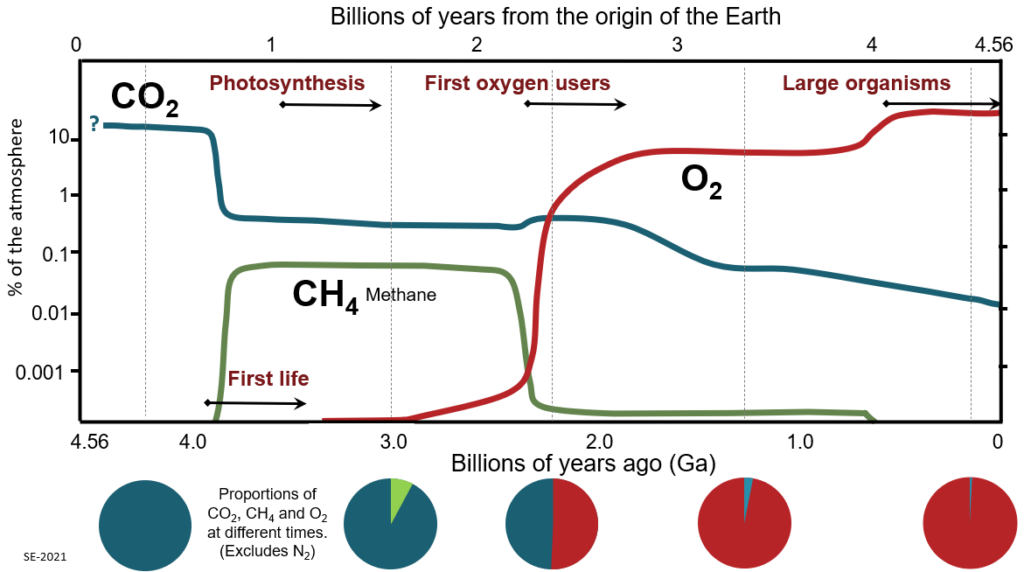
Life on Earth may have evolved in a sea-floor volcanic region close to scalding submarine hot springs (see Chapter 2), or in a shallow pool that was subject to repeated wetting and drying,[6] or in some othe
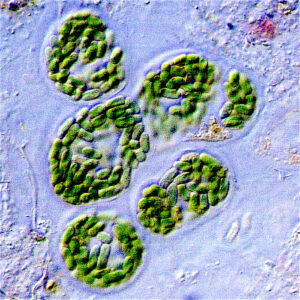
r environment with a source of heat and some useful molecules. In any case, the Earth’s atmosphere at the time was rich in carbon dioxide and had no free oxygen at all. Free oxygen (such as the O2 in our present atmosphere, and unlike the oxygen in CO2 or H2O) would have been a deadly poison to the earliest microorganisms, just as it is today to some of the things that thrive in the dark and damp places in our bodies and our homes, and in boggy environments around us. Many early life forms were methanogens—meaning that they produced methane—and so methane levels in the atmosphere increased as life started to thrive. This contributed to the warming because methane is a potent greenhouse gas.
Around 3.5 Ga (see Box 3.1) microorganisms developed the ability to use the sun as an energy source. The first of these were bacteria[7] (possibly cyanobacteria, a.k.a. blue-green algae – see Figure 3.1.4) and they had a distinct advantage over other life forms of the day in that they didn’t have to rely on energy from volcanic hot springs or from chemical sources: they could live virtually anywhere that the sun’s light could reach. The photosynthetic process involves consumption of carbon dioxide and release of oxygen, but the proportion of free oxygen in the atmosphere remained very low for almost another billion years. That’s because any oxygen produced by photosynthetic organisms was first used up in chemical reactions with abundant elements like iron, or gases like methane, and was also consumed through the decay of dead organic matter.
Box 3.1 What is a Ga?
The geological shorthand for describing time in the distant past involves the terms Ga, Ma and ka, where “a” stands for annum (year) and G, M and k stand for billion, million and thousand respectively. 3.5 Ga means “3.5 billion years ago”, 215 Ma means “215 million years ago”, and 14 ka means “14,000 years ago”.
All of these terms include that “years ago” clause, meaning that they are only used for indicating times past. They are not used to describe a span of time, so we can’t say “the dinosaurs lived for 149 Ma”. Instead, we have to say: “the dinosaurs lived for 149 million years”. It’s a bit like time of day notation. You can say: “my Environmental Geology class is at 2 pm”, but you cannot say: “the class is 2 pm long”. It’s just “2 hours long”.
Free oxygen first started to accumulate in our atmosphere around 2.4 Ga, initially in very small amounts. We call this transition the “oxygen crisis” because it led to extinction for many organisms. On the other hand, it really was the beginning of the beginning for organisms like us because the oxygen crisis appears to have pushed some existing organisms to evolve cells with a nucleus. Such organisms are eukaryotes, and they are our ancestors.
As more and more oxygen was produced it continued to react with methane (because methane and oxygen quickly react to form carbon dioxide and water) and the methane level dropped.[8] Since methane is a much more powerful greenhouse gas than carbon dioxide, the climate cooled dramatically, triggering an extensive period of glaciation starting around 2.3 Ga—the Huronian glaciation.[9] That was followed by a very warm period, and then the climate levelled out a bit, and there is no evidence of glaciation on Earth for another 1.6 billion years.
So, to summarize, it was relatively warm during most of the Earth’s first few billion years, despite a cooler sun. That’s because greenhouse gas levels were much higher than they are now. But there’s still a paradox here because life has evolved and flourished in liquid water (not frozen and not boiled off into space) for four billion years. How could the Earth have maintained a reasonable temperature throughout its history, a temperature comfortable enough (notwithstanding some ups and downs, such as the Huronian glaciation) for enough water to remain liquid? In other words, why has the Earth’s atmosphere changed as the sun has warmed, in such a way that a “Goldilocks” climate has existed here for four billion years?
Although we don’t fully understand why the Earth has been so habitable for so long, a key mechanism is the evolution of the atmosphere, and one of the drivers of that is photosynthesis. Various types of photosynthetic organisms—large and small, in the oceans and then later on land—have taken carbon dioxide from the atmosphere and released oxygen, converting the carbon to hydrocarbons and storing it in the rocks of the Earth’s crust (Figure 3.1.4). A parallel process, with a similar outcome, is the conversion of carbon from carbon dioxide into carbonate minerals. This is what most shelled organisms do to make their shells. Over time, as the sun has slowly warmed, life has used these two processes to reduce the greenhouse effect enough to keep the Earth from getting too hot.
The idea that life has controlled Earth’s climate to its own benefit forms the basis of the Gaia theory, first proposed by James Lovelock in 1972[10] and expanded upon by Lovelock and Lynn Margulis in 1974.[11] According to Lovelock and Margulis, the Earth and the living organisms on it form a self-regulating system that ensures that conditions remain suitable for life to persist, and they have been able to do so even as the system’s main source of energy (the sun) has slowly changed in intensity. This self-regulation proceeds through various types of biological processes and climate feedbacks. (There’s more on climate feedbacks in Box 3.2, below.)
Don’t worry, this is not a sinister conspiracy on the part of the Earth’s organisms to control the climate, it is just a result of natural feedbacks that have led to conditions being reasonable for the life present at any one time, or for new types of organisms to evolve that can take advantage of changing conditions. The more important thing is that carbon has been stored in Earth’s crust as a way of getting it out of the atmosphere to keep the climate reasonable, and we—just another lifeform—are upsetting that balance by removing a lot of that carbon from the crust and using it to drive our cars.
Box 3.2 Climate Feedbacks
A climate feedback is any process that can either amplify or dampen a climate forcing effect. A simple example is melting snow. When the temperature warms and enough snow melts to expose whatever is underneath it (e.g., bare ground or vegetation) the albedo at that location is decreased. As a result, more light can be absorbed and so the local area warms up more, and so more melting takes place and more light is absorbed, and so on. That’s an example of a positive feedback. It will keep working in that way until there’s no more snow to melt in that area. The following is a summary of some of the important feedbacks that have been amplifying climate changes—some of them for billions of years.
Feedback | Mechanism (as climate warming takes place) | Pos/neg |
Sea ice (or lake ice) | Sea ice melts to reveal open water. The albedo decreases, more solar energy is absorbed and so there is more melting. | Positive |
Snow and glacial ice | Snow and ice melt to reveal bare ground or vegetation, the albedo decreases, more solar energy is absorbed and so there is more melting. | Positive |
Water vapour | Warm air can hold more water vapour and that leads to more warming because water vapour is a GHG, although the effect is complicated by the cloudiness factor. | Positive |
Carbon dioxide solubility | The capacity of the oceans to absorb carbon dioxide decreases with increasing temperature and so, as ocean water warms, more of the huge ocean reservoir of CO2 is released into the atmosphere, producing more warming. | Positive |
Methane and CO2 in permafrost | Warming leads to melting of permafrost releasing stored methane and CO2 into the atmosphere, and so more warming. | Positive |
Vegetation growth (CO2) | The higher CO2 level that led to warming enhances plant growth which consumes more CO2, thus moderating the CO2 increase. | Negative |
Vegetation growth (albedo) | Enhanced vegetation growth makes a surface darker, so more solar energy is absorbed, leading to more warming. | Positive |
Vegetation distress | Vegetation may become distressed by warming so less CO2 is consumed and there is more warming. (Where cooling causes vegetation distress the feedback may be negative, as less CO2 is consumed.) | Positive |
Wildfire | Warming and regional drought increase the potential for wildfires, which result in CO2 and particulate emissions, and reduced CO2 consumption until the forest starts to regrow. | Positive |
Most of these feedbacks work just as well in reverse during a period of climate cooling. For example, as the climate cools, more snow (and perhaps glacial ice) will accumulate in some regions, increasing the albedo and leading to more cooling. Or, with cooling, more carbon dioxide gets dissolved in the oceans, and so the greenhouse effect is reduced, and cooling is enhanced.
An alarming thing about feedbacks is that almost all of them are positive, and so there is a strong tendency for a little bit of warming to be amplified into a lot warming, and vice versa with cooling. In fact, if that wasn’t the case it’s likely that many of the dramatic climate changes that have taken place in the past would never have happened. For example, we might not have had multiple glaciations over the past million years, or we might have had nothing but glaciation for the past million years—with half of North America still covered in ice!
Media Attributions
- Figure 3.1.1 Steven Earle, CC BY 4.0, after Solar Life Cycle image, public domain, by Oliver Beatson, via Wikimedia Commons, https://commons.wikimedia.org/wiki/File:Solar_Life_Cycle.svg
- Figure 3.1.2 Steven Earle, CC BY 4.0; based on information in Ribas, I. (2009). The sun and stars as the primary energy input in planetary atmospheres. Proceedings of the International Astronomical Union, 5(S264), 3–18. https://doi.org/10.1017/S1743921309992298
- Figure 3.1.3 Steven Earle, CC BY 4.0, after Nisbet E., Fowler C., (2011). The evolution of the atmosphere in the Archaean and early Proterozoic. Chinese Science Bulletin, 56, 4−13. https://doi.org/10.1007/s11434-010-4199-8; and Large, R., Mukherjee, I., Gregory, D. et al. (2019). Atmosphere oxygen cycling through the Proterozoic and Phanerozoic. Mineral Deposita 54, 485–506. https://doi.org/10.1007/s00126-019-00873-9
- Figure 3.1.4 Cyanobacteria from Guerrero Negro, NASA public domain image, via Wikimedia Commons, https://commons.wikimedia.org/wiki/File:Cyanobacteria_guerrero_negro.jpg
- The history of the Sun (and other stars) is summarized by David Taylor of Northwestern University, http://faculty.wcas.northwestern.edu/~infocom/The%20Website/index.html ↵
- Luminosity is a measure of the amount of energy emitted by the Sun, and that is directly proportional to how much solar energy is received on the surface of the Earth. ↵
- Virtually all references to the Earth’s surface “temperature” in this volume refer to the mean annual temperature (MAT), which is the average temperature over every square kilometre of the Earth—including the tropics and the poles, the oceans and the land—and also averaged over an entire year. ↵
- There are no fossils as old as 4 Ga, but there is chemical evidence of life in rocks that old, specifically carbon deposits that have the isotopic signature of being formed by living organisms. The oldest undisputed evidence of life is in the form of fossils dating to about 3.5 Ga. (Tashiro, T., Ishida, A., Hori, M., Igisu, M., Koike, M., Méjean, P., Takahata, N., Sano, Y., & Komiya, T. (2017). Early trace of life from 3.95 Ga sedimentary rocks in Labrador, Canada. Nature, 549(7673), 516–518. https://doi.org/10.1038/nature24019) ↵
- The geological time scale is described in Chapter 1. You can find a copy of the full chart on the International Commission on Stratigraphy website (https://stratigraphy.org/chart). ↵
- Becker, S., Feldmann, J., Wiedemann, S., Okamura, H., Schneider, C., Iwan, K., Crisp, A., Rossa, M., Amatov, T., & Carell, T. (2019). Unified prebiotically plausible synthesis of pyrimidine and purine RNA ribonucleotides, Science, 366(6461): 76-82. https://doi.org/10.1126/science.aax2747 ↵
- Blankenship, R. E. (2010). Early evolution of photosynthesis. Plant physiology, 154(2), 434–438. https://doi.org/10.1104/pp.110.161687 ↵
- The reaction goes like this: CH4 + 2O2 -> CO2 + 2H2O. One molecule of methane reacts with two oxygens to produce one carbon dioxide and two molecules of water. In other words, methane is being oxidized to carbon dioxide. Of course, carbon dioxide is also a greenhouse gas, but it is only about 1/20th as potent a warming agent as is methane. ↵
- Evidence from ancient rocks suggests that the Huronian glaciation lasted for at least 40 million years (from 2.29 to 2.25 Ga). It is thought to have been widespread, based on glacial evidence in rocks of that time from Canada, USA, Europe, South Africa, India, Australia, and Brazil, and may have affected low latitudes as well as polar regions. The oceans may have been mostly frozen over. Tang, H., & Chen, Y. (2013). Global glaciations and atmospheric change at ca. 2.3 Ga. Geoscience Frontiers, 4(5): 583–596. https://doi.org/10.1016/j.gsf.2013.02.003. ↵
- Lovelock, J.E. (1972). Gaia as seen through the atmosphere. Atmospheric Environment, 6, 579-580. ↵
- Lovelock, J., & Margulis, L. (1974). Atmospheric homeostasis by and for the biosphere: the Gaia hypothesis, Tellus, 26(1-2), 2-10. ↵