10.5 Clay Minerals
Clay Mineral Structures
Clay minerals are comprised of silica tetrahedra and alumina octahedra, which are illustrated on Figure 10.5.1. As we’ve seen in Chapter 2 (Figure 2.1.5), a silica tetrahedron is a silicon ion surrounded by four oxygen ions. Planes drawn through lines connecting the oxygens atoms define a tetrahedral (four-surfaced) shape. An alumina octahedron is an aluminum ion surrounded by six oxygen or hydroxyl ions. Planes drawn through lines connecting the oxygens and hydroxyl atoms define an octahedral (eight-surfaced) shape.
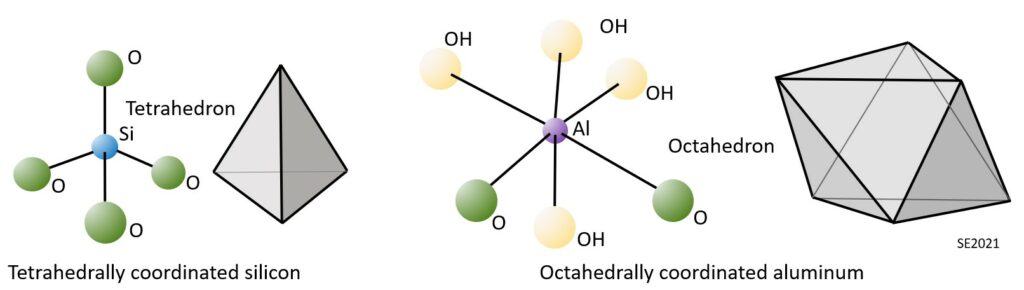
The simplest clay mineral is kaolin. Each “sheet” within the kaolin structure is comprised of a silica tetrahedral layer and an aluminum octahedral layer (Figure 10.5.2). The combination of one tetrahedral layer and one octahedral layer makes this a 1:1 layer silicate. For simplicity, it may be useful to describe this as a T-O structure (1 tetrahedral layer and 1 octahedral layer). This structure is also found in the mineral serpentine, in which magnesium substitutes for aluminum in the octahedral sites.
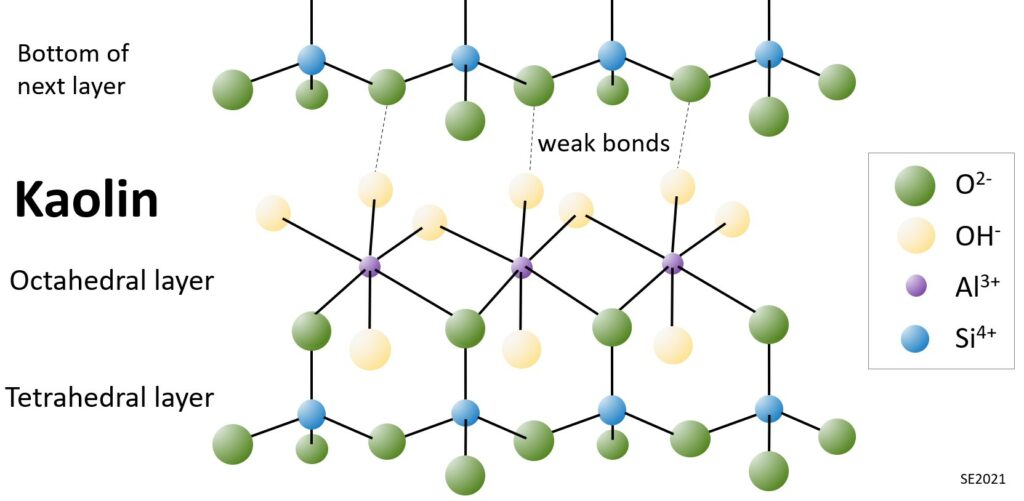
The structure of illite is more complicated than that of kaolin. In this case each “sheet” within the structure is comprised of an aluminum octahedral layer sandwiched between two tetrahedral layers (one “right side up” and the other “up-side down”) (Figure 10.5.3). Illite also has potassium ions situated at specific sites between the sheets. The combination of two tetrahedral layers surrounding one octahedral layer is known as a 2:1 layer silicate. We can also describe this as a T-O-T structure. Some other T-O-T clays include smectite, talc and chlorite.
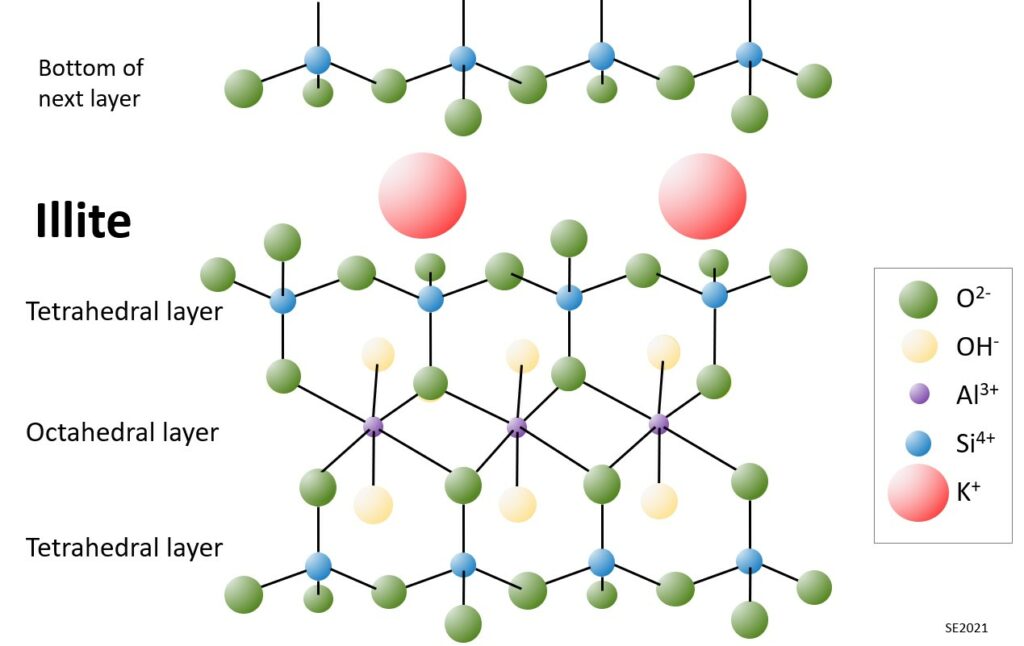
The potassium cations (K+) of illite are held in place because the upper and lower surface of each layer is saturated with oxygen ions (O-2) giving these surfaces a consistent negative charge. These negatively charged surfaces are one of the fundamentally important features of clay minerals, because such surfaces are attractive to positively charged ions, such as heavy metals, or some organic pollutants. Not only do clays have these attractive surfaces, but they have very large surface areas. It is estimated that a cubic centimetre of clay has a reactive surface of around 2800 square metres, which is equivalent to the area of a football field!
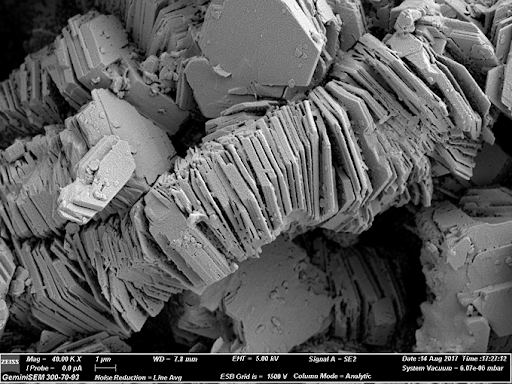
Some kaolin crystals are shown Figure 10.5.4. The kaolin plates are stacked up in loosely defined strands. Each visible plate is between 1/10th and 1/5th of a micron thick, but each of these is made up of hundreds to thousands of the T-O layers illustrated in Figure 10.5.2. There is a significant amount of empty space between the plates.
Although there are many dozens of different clay minerals, there are just a handful that are important for us to be aware of here, and these are summarized in Table 10.3. The first thing to note is that there are only two 1:1 (T-O) clay minerals in this list, kaolin and serpentine, while all of the others are 2:1 clays (T-O-T). Most of these 2:1 clays have magnesium and/or iron in them, and so can be considered ferromagnesian silicates. The only non-ferromagnesian clays listed are kaolin, pyrophyllite and Illite, although Illite can also have small amounts of magnesium, and the glauconite variety has iron. The important point here is that while kaolin, pyrophyllite and Illite are typically derived from alteration of minerals like feldspar and muscovite, most of the other clays are derived from alteration of minerals like olivine, pyroxene, amphibole and biotite.
Clay Mineral | Type | Typical Chemical Formula | Variations and (other names) |
Kaolin | 1:1 | Al2Si2O5(OH)4 | kaolinite, dickite, halloysite, nacrite |
Serpentine | 1:1 | Mg3Si2O5(OH)4 | antigorite, chrysotile (asbestos), lizardite |
Illite | 2:1 | K0.65Al2.0(Al0.65Si3.35O10)(OH)2 | glauconite, (hydromuscovite, K-deficient muscovite) |
Pyrophyllite | 2:1 | Al2Si4O10(OH)2 | |
Smectite | 2:1 | (Na, Ca)0.33(Al, Mg)2(Si4O10)(OH)2 ∙ nH2O | montmorillonite (bentonite), saponite, nontronite |
Vermiculite | 2:1 | (Mg, Fe2+, Fe3+)3 ((Al, Si)4O10)(OH)2 ∙ 4H2O | |
Talc | 2:1 | Mg3Si4O10(OH)2 | |
Chlorite | 2:1 | (Mg, Fe)3(SI, Al)4O10(OH)2 ∙ (Mg, Fe)3(OH)6 | clinochlore, pennantite, chamosite, sudoite |
Formation of Clay Minerals
Primary Silicates | Typical Clay Minerals That Will Form Under Weathering Conditions |
Olivine | smectite |
Amphibole & pyroxene | smectite, talc, vermiculite & chlorite |
Plagioclase feldspar | kaolin (especially halloysite or kaolinite) |
Potassium feldspar | kaolin (and illite less commonly) |
Biotite | vermiculite, kaolin |
Muscovite | Tends to be generally resistant to weathering but can convert to illite |
The clay mineral products listed Table 10.4 are specifically those that form under weathering conditions, which generally means temperatures under 40° C, atmospheric pressure, and water that has low levels of dissolved ions and close to neutral pH.
Some of the clay-mineral transformations that can take place within sediments as they undergo progressively greater burial beneath other sediments are illustrated on Figure 10.5.5. The assumption here is that the sediments already include some clay minerals, especially the low-temperature clays smectite and kaolin produced during weathering in the sediment source area. Starting at around 100° C, the smectite might first be altered to a mineral with mixed or alternating layers of smectite and illite. The mixed-layer clays may be altered to chlorite and illite at around 150° C, with the illite being altered to muscovite at over 200° C. Any kaolin originally present in the sediment as kaolinite or halloysite might first get transformed to the higher temperature polymorphs (dickite or nacrite) and then to illite, and eventually to either chlorite or muscovite.
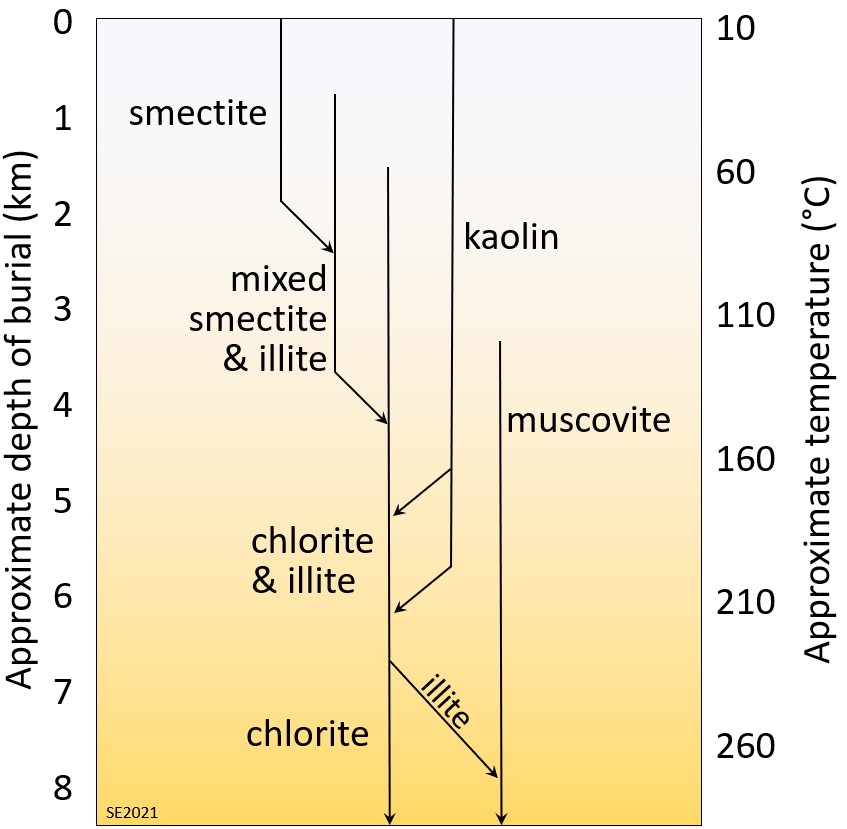
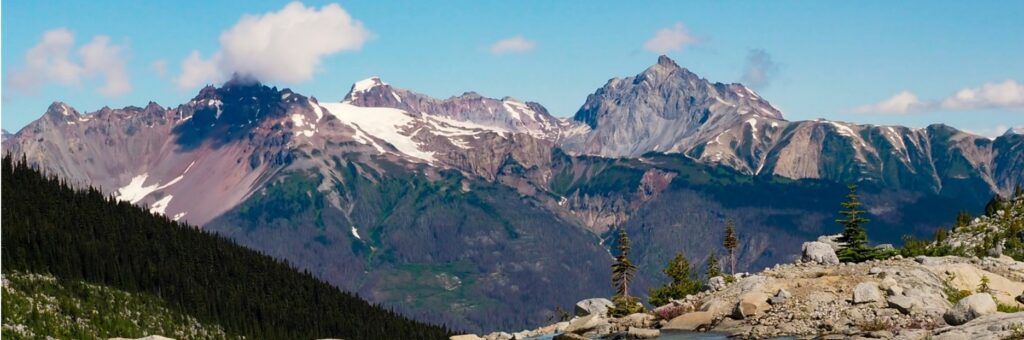
Exercise 10.5 Clay Mineral Origins
Some clay minerals are listed below. Indicate the environment (e.g., weathering, diagenesis, hydrothermal alteration) in which it likely formed, and a possible precursor mineral.
Mineral | Likely Environment of Formation and Possible Precursor |
Halloysite | |
Mixed-layer clay | |
Serpentine | |
Illite | |
Dickite |
Exercise answers are provided Appendix 2
Properties of Clay Minerals
The tetrahedra and octahedra that make up clay minerals have negatively charged ions (anions) on their outsides (either O2- or OH–) making the surfaces of the individual layers negatively charged, and therefore attractive to positively charged ions (cations) in solution. Most metals exist as cations and many organic pollutants have positive charges, and so clay minerals are efficient scavengers of environmental pollutants, and can be used as barriers to prevent dispersal of contaminants and also in environmental rehabilitation projects. Different clays have different capacities to absorb cations (known as “cation exchange capacity”), and some of these are listed in Table 10.5.[2] Smectite has a much higher cation exchange capacity than other clays because cations can get onto the sites in between the molecular layers within a crystal, as opposed to just the outside surfaces of the crystals.
– | Effective Surface Area in m2/g | – | Cation Exchange Capacity |
Mineral | Interlayer | External | Meq/g* |
Kaolin | 0 | 15 | 1 to 10 |
Chlorite | 0 | 15 | <10 |
Illite | 5 | 15 | 10 to 40 |
Smectite | 750 | 50 | 80 to 150 |
The smectite clays have a 2:1 structure similar to that of illite (Figure 10.4.3) but the interlayer cations are typically either sodium, calcium or magnesium (instead of potassium). This means that the layers are a little further apart than in illite, and for that reason smectites have a unique ability to absorb water molecules in the interlayer sites. This is especially true for sodium smectites, which can absorb up to 18 layers of water in between the sheets, and thereby will expand or swell dramatically when wet. Some swelling clay is shown on Figure 10.5.8. The clay is present within a depression and was wet. On drying it shrank in the typical mudcrack pattern. Swelling clays have a number of important industrial and domestic uses, but they also have some serious geological implications. A swollen wet smectite is even weaker than a dry one, so can weaken slopes significantly, and bodies of swollen clay can distort the materials around them, also potentially contributing to slope failure or problems for building or road foundations.
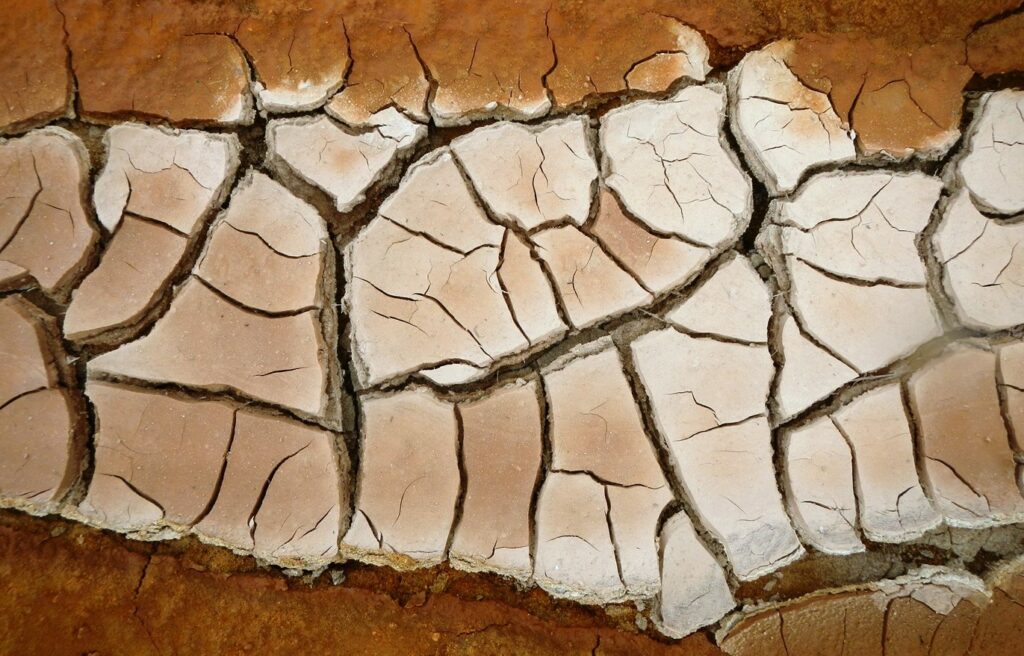
Vermiculite will also swell slightly when wet, but, unlike other clays it will expand dramatically on heating (Figure 10.5.9). When heated to 500 to 800° C the water trapped between the layers will boil and push the layers apart increasing the volume dramatically. Expanded vermiculite has many uses, including as a growing medium, insulation, brake linings, and fireproof panels.
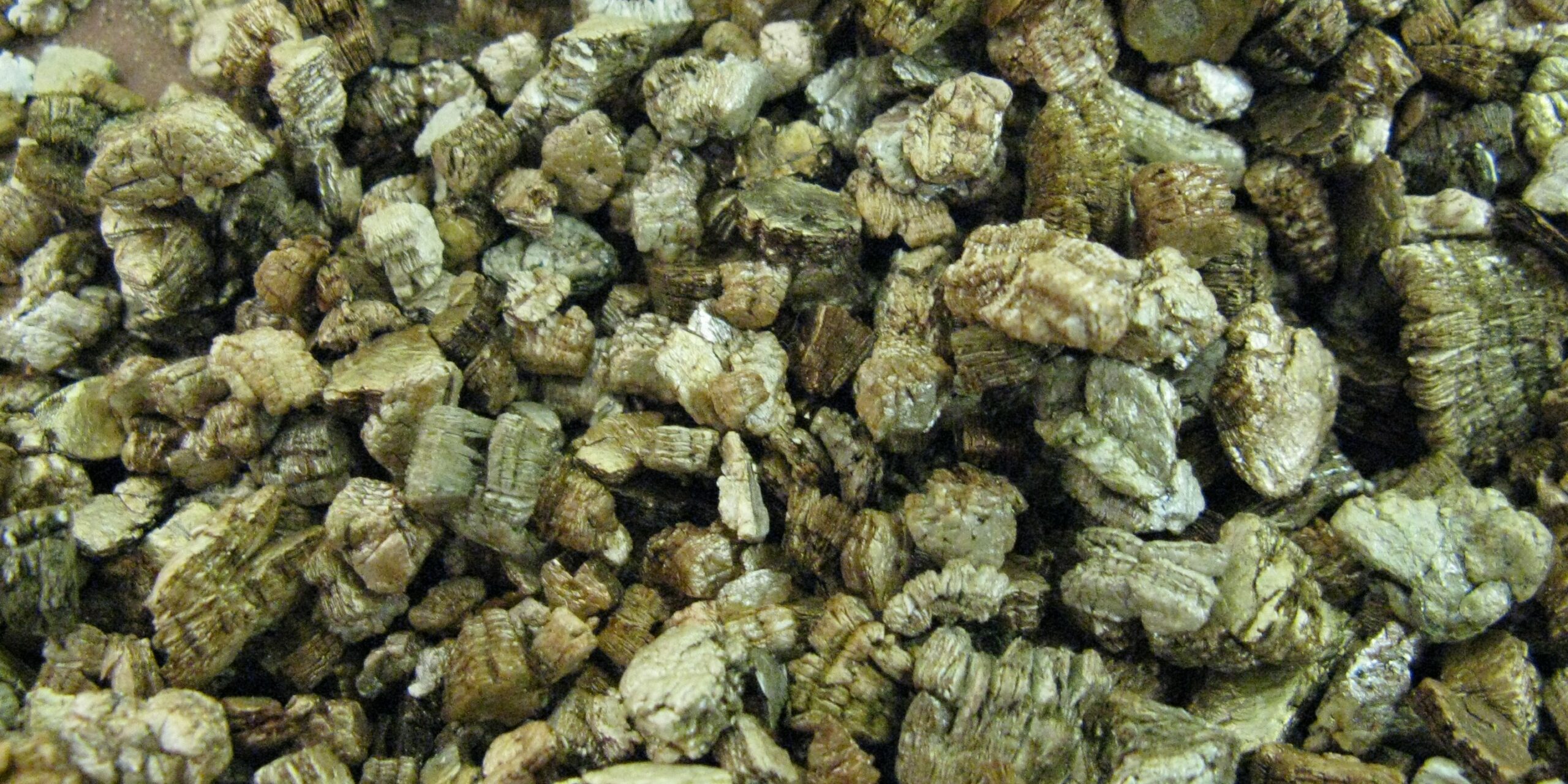
Exercise 10.6 Find Some Clay in Your Neighbourhood
Clay Minerals and Earth Systems
- Clay minerals may have played a role in the initial evolution of life from organic chemicals because the regular structure of the clays could have acted as a template for the assembly of organic molecules,
- Conversion of silicate minerals to clay consumes atmospheric CO2 and so has climate implications,
- Clay minerals accumulate trace elements that later become available to plants and microorganisms,
- Clay minerals accumulate trace elements that may eventually get concentrated into mineral deposits,
- Clay minerals can reduce rock strength and so contribute to erosion and slope failure,
- Clay minerals suspended in water or as clouds of dust can be vehicles for the transfer of trace and major elements from land into the ocean (See Figure 10.5.10), and
- Clay minerals are the vehicles for the transfer of water from subducted oceanic crust into the mantle, leading to magma formation (via flux melting) and volcanism.
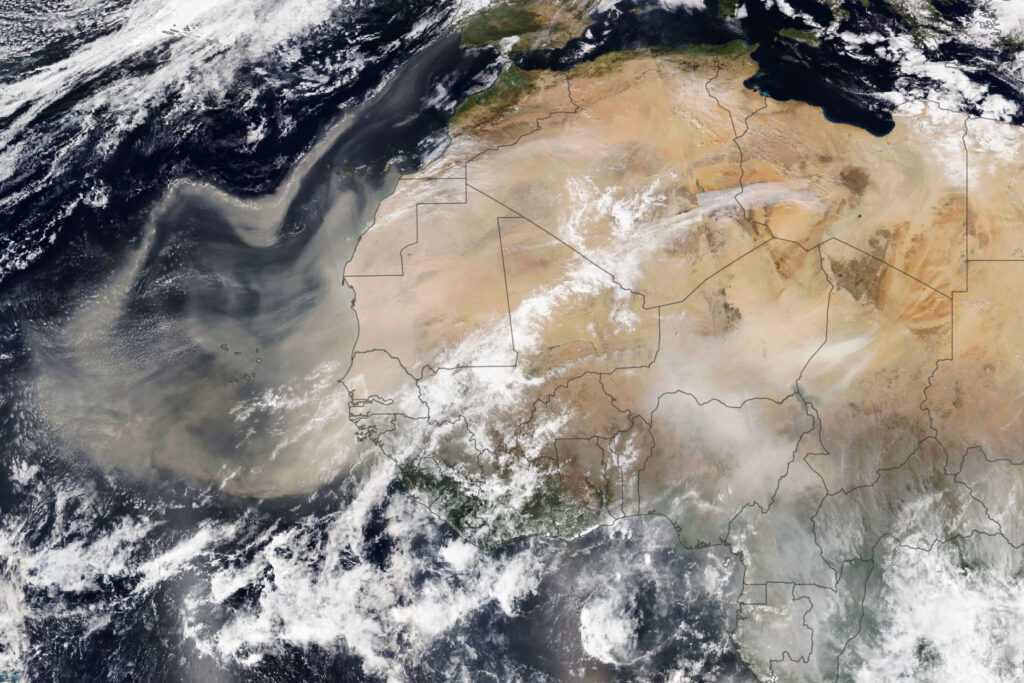
- Figure 10.5.1 Steven Earle, CC BY 4.0
- Figure 10.5.2 Steven Earle, CC BY 4.0
- Figure 10.5.3 Steven Earle, CC BY 4.0
- Figure 10.5.4 Plates of the Clay Mineral Kaolin from ACEMAC Nano Scale Electron Microscopy and Analysis Facility, University of Aberdeen by GSoil, CC BY 3.0, https://www.abdn.ac.uk/business-info/facilities-and-expertise/acemac-nano-scale-electron-microscopy-and-analysis-facility-846.php#panel861
- Figure 10.5.5 Steven Earle, CC BY 4.0, after Figure 7.13. In Prothero, D. and Schwab, F. (2004). Sedimentary geology: An introduction to sedimentary rocks and stratigraphy (2nd ed.). Freeman and Co.
- Figure 10.5.6 Photo by Isaac Earle, CC BY 4.0
- Figure 10.5.7 Serpentinite by James St. John, CC BY 2.0, via Flickr, https://www.flickr.com/photos/jsjgeology/16940796272
- Figure 10.5.8 Photo by Steven Earle, CC BY 4.0
- Figure 10.5.9 Vermiculite by KENPEI, 2008, CC BY-SA 2.1 JP, via Wikimedia Commons https://commons.wikimedia.org/wiki/File:Vermiculite1.jpg
- Figure 10.5.10 Africa Dust from NASA, public domain, https://eoimages.gsfc.nasa.gov/images/imagerecords/147000/147952/africadust_virs_202149_lrg.jpg
- There are several different forms of the mineral kaolin. The best known is kaolinite. Others include halloysite, which forms at low-temperatures, and dickite and nacrite at higher temperatures. ↵
- The data in Table 10.5 are based on information in Wilson, M. (2004). Weathering of the primary rock-forming minerals, processes, products and rates. Clay Mineralogy, 39(3), 233-66. doi:10.1180/0009855043930133; and in Deer, W., Howie, R., and Zussman, J. (1991). An introduction to the rock-forming minerals (2nd ed). Longman. ↵